Taiwan Operational Meteorology-Ocean Observing Network (TOPMOON)
People
- Principal Investigators :
- Sen Jan
- Professor at Institute of Oceanography, National Taiwan University
- Je-Yuan Hsu
- Assistant Professor at Institute of Oceanography, National Taiwan University
- Wei-Yu Chang
- Associate Professor at Department of Atmospheric Sciences, National Central University
- Ming-Huei Chang
- Professor at Institute of Oceanography, National Taiwan University
- Yu-Hsin Cheng
- Assistant Professor at Department of Marine Environmental Informatics, National Taiwan Ocean University
- Dong-Lin Li
- Assistant Professor at Department of Electrical Engineering, National Taiwan Ocean University
- Project Manager :
- I-Chang Liu
- Institute of Oceanography, National Taiwan University
- Project Coordinator :
- Wei-Ting Tien
- Institute of Oceanography, National Taiwan University
A short description for TOPMOON
The establishment of a meteorology-ocean observing network is motivated by the long-term lack of a systematic ocean observing network in the sea surrounding Taiwan, and is urgent to improve the infrastructure of fundamental ocean and meteorological research and outposts for disaster mitigation using real-time data transmission technology. We propose a four-year work plan to achieve the objectives of TOPMOON, including supporting ocean-atmosphere scientific research, advancing marine disaster early warning ability, and reducing social impacts from extreme oceanic events. Operational data buoy system, vessel-mounted meteorological radar, real-time data transmission for deep sea instruments, concurrent underwater gliders observation ability, and value-added multi-satellite data will be built along with the project with supports of existing observational resources and technologies from the academic community in Taiwan. Oceanic talent cultivation and connection to the global ocean observing system (GOOS) will be beneficial from the operation of TOPMOON.
Keywords: prototype operational ocean meteorology value-added
Background
Located in the junction of the Eurasia and Philippine Sea plates and in the western boundary of the North Pacific (Fig. 1), Taiwan is beneficial from the biological and non-biological resources in the surrounding seas, and also suffers from typhoons, earthquakes, and global warming from the ocean. In addition to energetic geological activities (Sibuet et al., 2005; among others), the surrounding seas of Taiwan are influenced by the western boundary current of the North Pacific, Kuroshio, westward-propagating mesoscale eddies (e.g., Chelton et al., 2007; Yang et al., 2013; Cheng et al., 2014, 2017; Chang et al., 2015; Jan et al., 2017; Chang et al., 2018) and typhoons (e.g., Jan et al., 2017; Yang et al., 2019), resulting in a sensitive and complicated marine environment. From the management of marine resource and the mitigation of marine disaster points of view, a complete knowledge of oceanography based on comprehensive field observations is the key to the success (e.g., Cheng and Chang, 2018). On the land of Taiwan, we have established complete earthquake monitoring and meteorological observing networks. Comparing with the two networks, similar observations are still meager in the ocean, particularly, east of Taiwan. The long-term lack of a sustainable ocean observing network in the sea surrounding Taiwan motivates the idea of this integrated project. The establishment of the operational four-dimensional meteorology-ocean observing network (TOPMOON) as an infrastructure of fundamental ocean and meteorological research is crucial to an outpost for disaster mitigation using real-time data transmission technology. The urgent need for establishing such an observing network for Taiwan is clear.
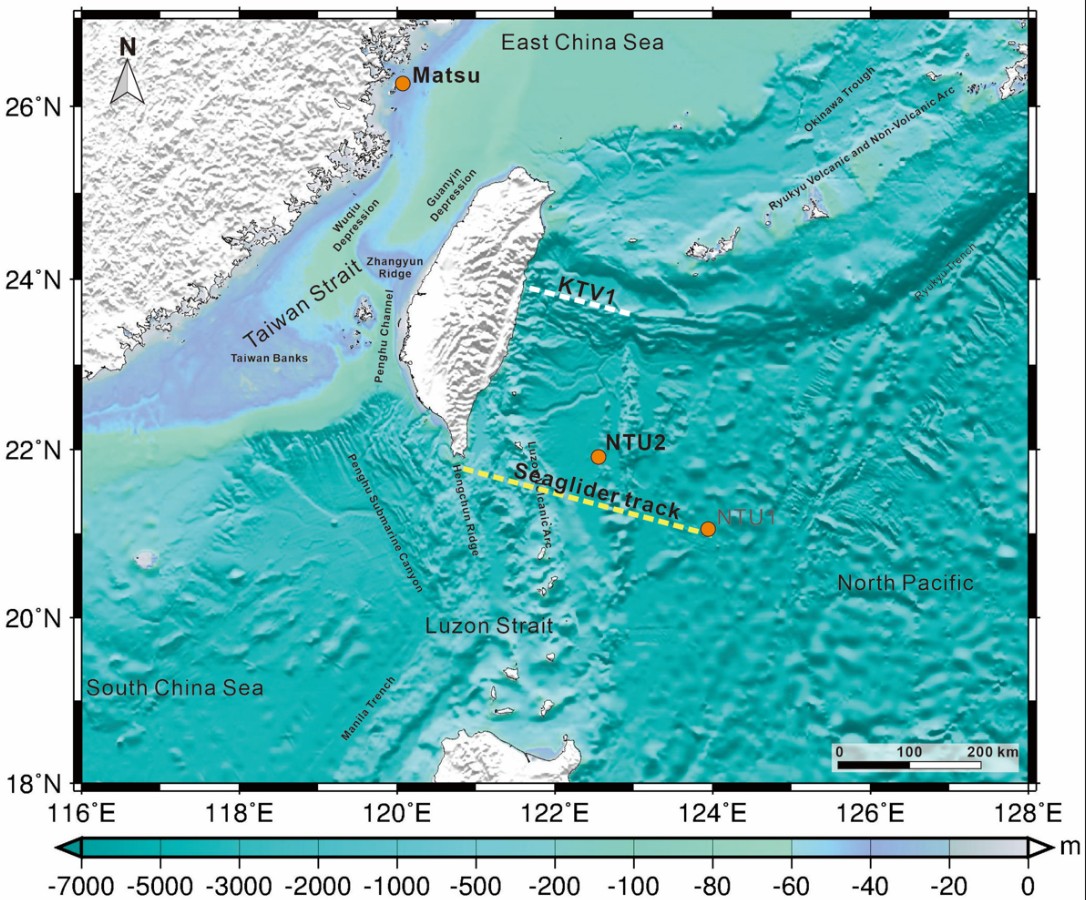
In the complicated dynamics between the atmosphere and ocean, the wind forcing at the air-sea interface, which can be modulated by surface waves, can significantly affect the upper ocean structure, and thereby sea surface temperature (SST) variations. More and more atmosphere-wave-ocean coupled models are thus proposed and operated for the daily weather forecast, such as ECMWF. Compared to the traditional atmosphere-ocean coupled models, simulating surface wave dynamics during the model runs can affect the momentum and thermal flux exchange. As an island located in the northwest Pacific, the ocean near Taiwan is a hot spot for the passage of typhoons from summer to autumn annually. Because the ocean is the energy source for most weather systems, including typhoons, assimilating in-situ atmospheric and wave measurements can directly enhance the model performance. Moreover, even if the storm wind does not directly cause the loss of human properties, strong waves and storm surges can travel a long distance and affect the fishery and coastlines around Taiwan. Therefore, establishing a long-term database including marine meteorology and surface waves can benefit model forecasts on the weather systems and sea state around Taiwan.
Goals
Tasks
The associated tasks for achieving these goals comprise the coordination of six principal investigators from National Taiwan University (NTU), National Central University (NCU), and National Taiwan Ocean University (NTOU) being responsible for the deployment, maintenance, and recovery of data buoys, strengthening of ship-based meteorological and wave observations, improvement of marine geophysical measurements, international cooperation of glider observations, and development of underwater acoustic modem for data transmissions and communications (see a conceptual diagram in Fig. 2). The main-project aims to create a platform for these principal investigators to work together and to perform and sharpen their expertise in these different disciplinary. This is also crucial for cultivating seagoing oceanographers of next generation. Doubtlessly, these mission-oriented tasks are fundamental (but not full) components in a meteorology-ocean observing network. The in-situ observations and real-time data transmission proposed by the project are important to improve the national disaster (particularly from the ocean) early warning ability and could be beneficial to the associated disaster mitigation. The observational data will be processed, quality-assured, and value-added in a project data website and in collaboration with Taiwan’s Ocean Data Bank. The field data obtained from TOPMOON at a ship-based long-term observation section across the Kuroshio KTV1 line, a fixed glider observing track, two long-term data buoys NTU2 and Matsu (Fig. 1), fundamental ocean bottom geophysical observations, etc. will be used to supplement associated scientific research in (but not limited to):
The data collected by TOPMOON will also be a basic state of the marine environment around Taiwan as well as western North Pacific for the assessment of impact from the climate change to the ocean. In addition to the research applications, the value-added products will be provided to the associated government agencies such as the Central Weather Bureau and National Science and Technology Center for Disaster Reduction of Taiwan in a timely mode.
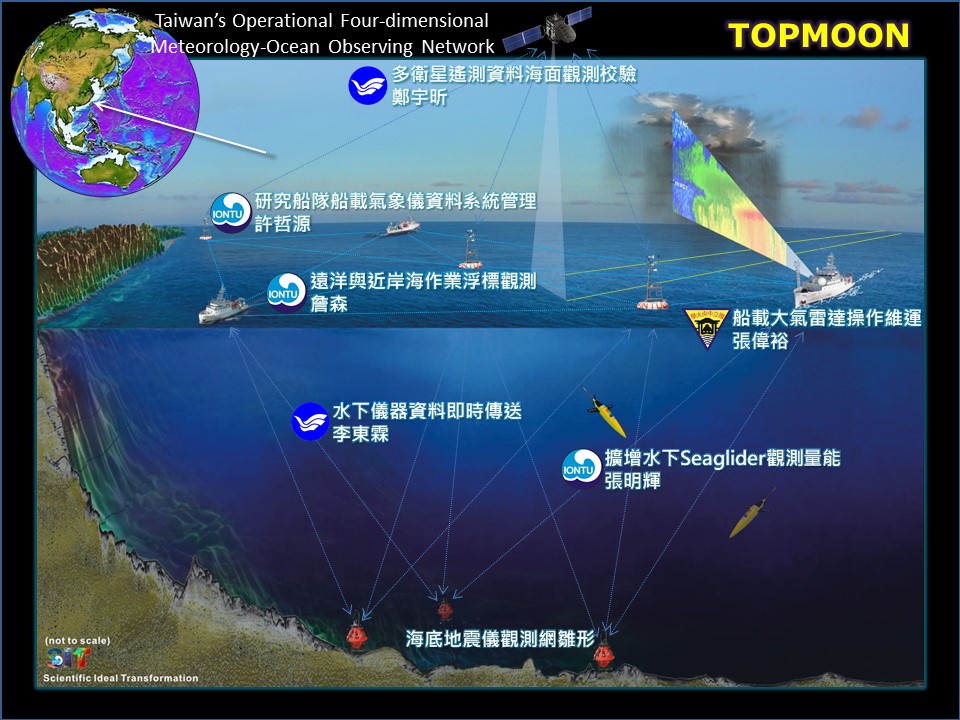
Expect achievements
Work plan
TOPMOON plans to build two operational data buoy systems, install vessel-mounted Doppler radar scanning system, conduct concurrent underwater gliders observations, validate satellite-derived sea surface wind and wind waves using the field data of this project, and establish real-time data transmission for deep sea instruments.
Support
References